Human cells have complex mechanisms to repair DNA damage, such as that caused by exposure to sunlight or chemical substances. If DNA is not properly repaired, however, it can lead to cancer. In fact, faulty DNA repair has been associated with the initiation and progression of all types of cancer and is often targeted in cancer treatment to stop uncontrolled cell growth. A better understanding of how cells naturally defend against DNA damage will allow for the development of better drugs to treat cancer. Dr. Hoitsma [HHMI Fellow] aims to investigate specialized proteins, known as chromatin remodelers, that make damaged DNA accessible for repair. This research will provide insight for the development of novel therapeutic strategies to target these critical pathways. Dr. Hoitsma received her PhD from University of Kansas Medical Center, Kansas City and her BS from South Dakota State University, Brookings.
All Cancers
Current Projects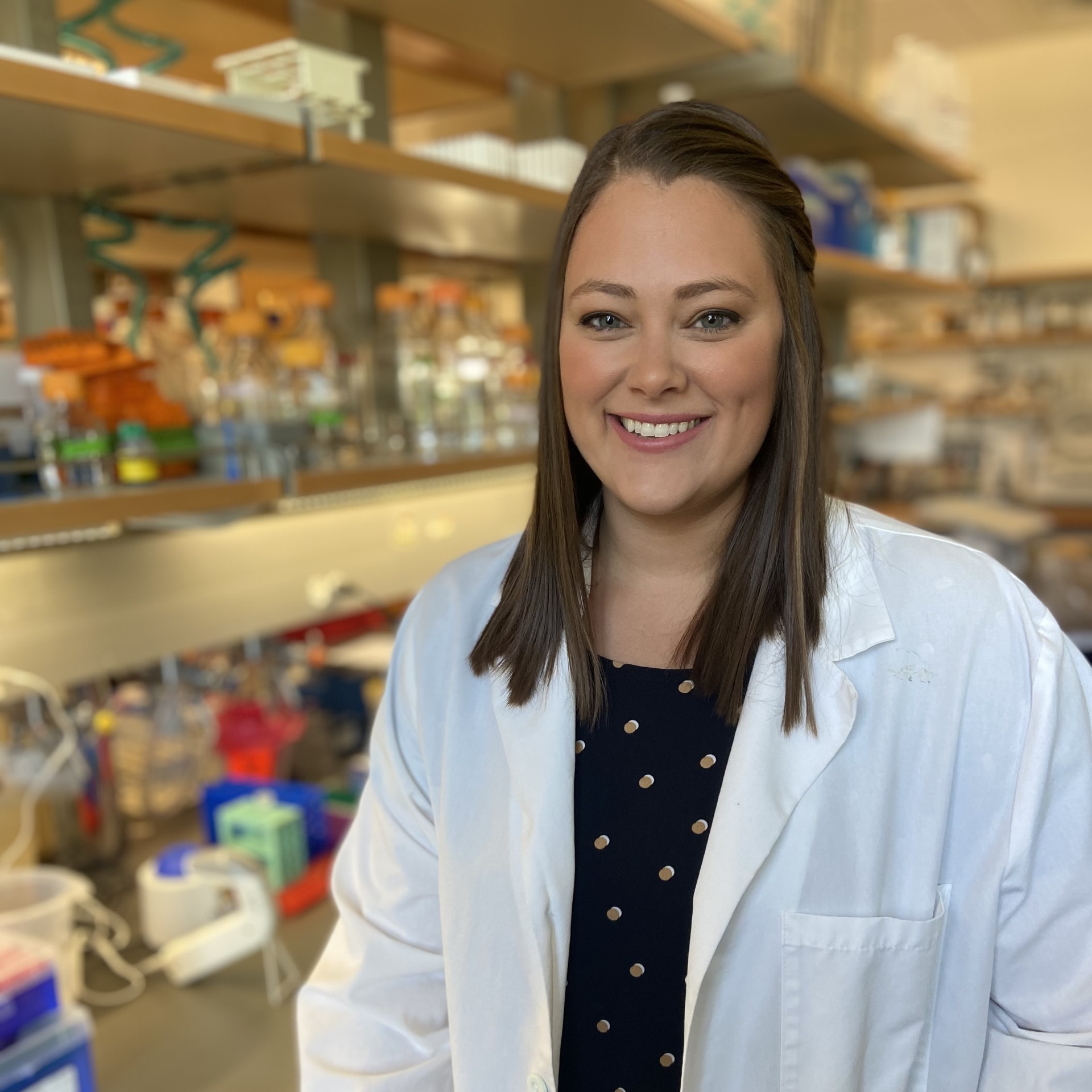
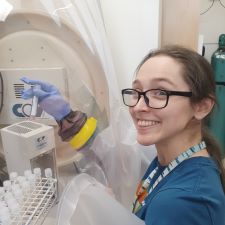
Immune checkpoint inhibitors, a type of cancer treatment that helps immune cells identify and kill tumor cells, have been a major breakthrough in the treatment of many cancer types. Unfortunately, not all patients respond to this immunotherapy. Dr. Hughes [Robert Black Fellow] is studying how gut microbes improve response to immune checkpoint inhibitors. The bacterium Akkermansia muciniphila lives in the gastrointestinal tract and has been shown to improve response to immune checkpoint inhibitors via poorly understood mechanisms. Dr. Hughes aims to discover how A. muciniphila improves response to cancer immunotherapies and to design microbe-based therapeutic strategies that will further enhance cancer immunotherapy responses. Dr Hughes received her PhD from UT Southwestern Medical Center and her BS from Baylor University.
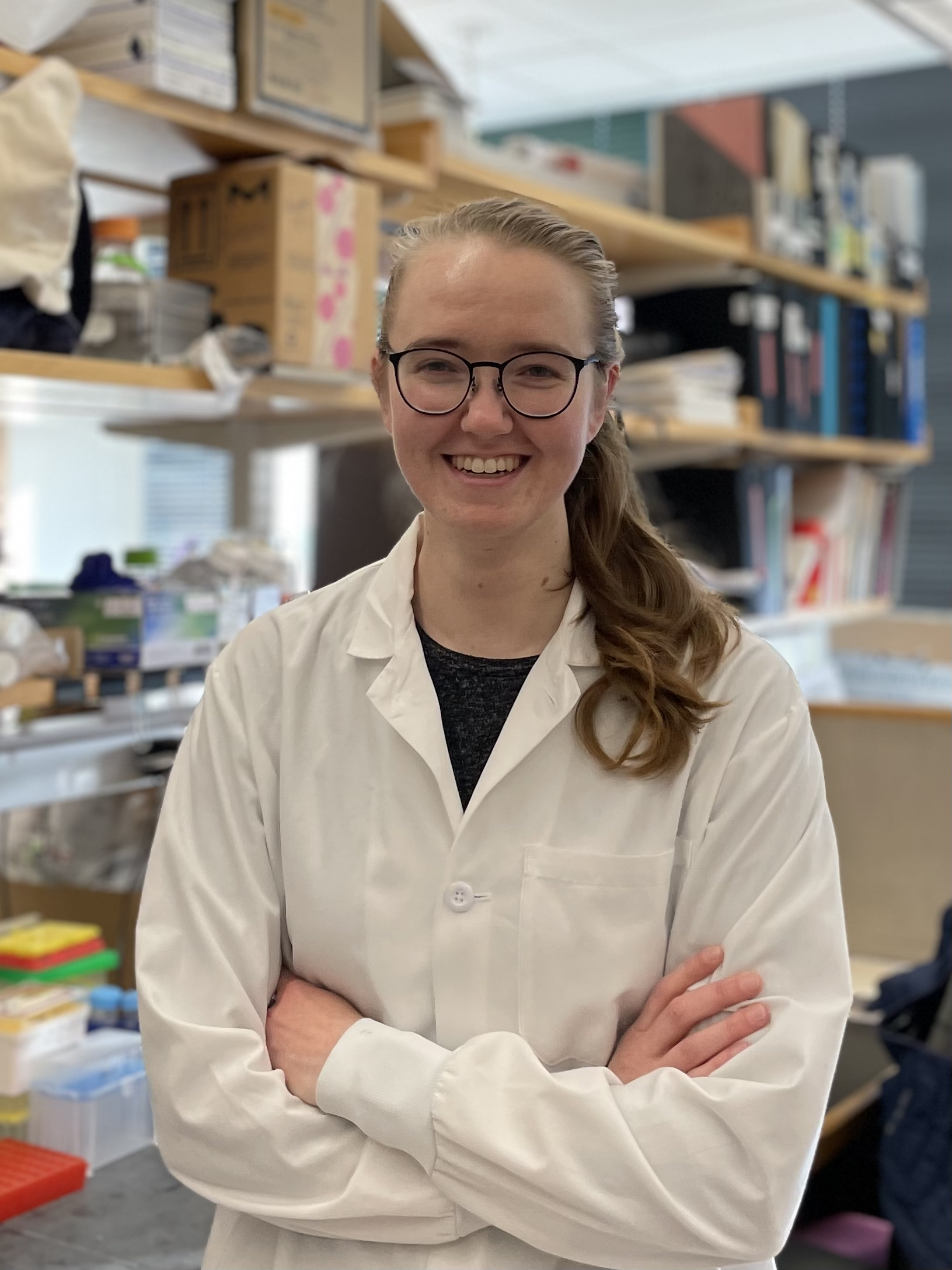
To prevent autoimmune attacks, T cells are screened in the thymus to ensure they do not react to self-derived antigens. Dr. Huisman [National Mah Jongg League Fellow] studies the thymus and, specifically, a population of cells called “thymic mimetic cells” that mimic other tissues, such as muscle or gut, and assist T cells in developing tolerance to diverse cell types. Dr. Huisman’s research focuses on understanding how thymic mimetic cells develop. This work may lead to improved understanding of thymus-mediated tolerance to tumors, novel therapeutic opportunities for manipulating mimetic cells to induce anti-tumor responses, and increased understanding of thymic tumors. Dr. Huisman received her PhD from Massachusetts Institute of Technology, Cambridge and her BS from University of Michigan, Ann Arbor.
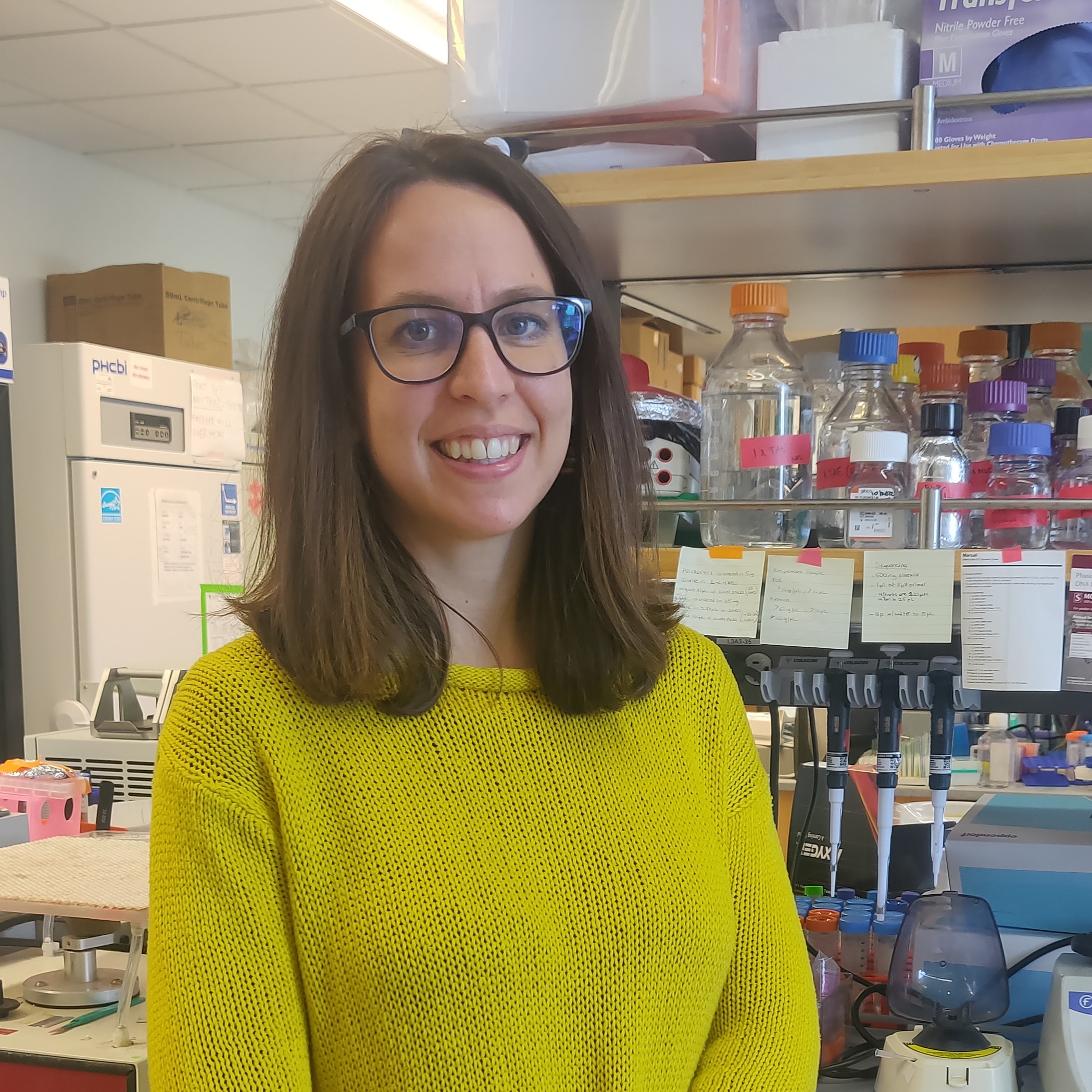
Epithelial to Mesenchymal Transition (EMT) is a crucial biological process that occurs during early development. It allows epithelial cells, which line the inner and outer surfaces of the body, to undergo a profound transformation in cellular identity and migrate and populate the embryo. Unfortunately, numerous cancer types exploit this mechanism, allowing cancer cells to detach from the tissue of origin and disseminate throughout the body, significantly worsening patients’ prognoses. Dr. Ichino [HHMI Fellow] is studying the process of developmental EMT with the goal of discovering novel ways to interfere with it in the context of cancer progression. Dr. Ichino’s research takes advantage of a lab-grown system that mimics the EMT and migration of neural cells. Using this system, she plans to study how EMT-promoting transcription factors orchestrate this global change in cellular identity, and how genetic variations can influence this process. Dr. Ichino received her PhD from University of California, Los Angeles and her MS and BS from San Raffaele University, Milan.

Successful immune responses against cancer require immune cells of various types to control each other’s proliferation, differentiation, and death. These interactions collectively constitute a set of intercellular signaling circuits. A fundamental challenge in cancer research is to understand the relationship between the architecture and functions of these circuits. Dr. Indana [HHMI Fellow] will quantitatively model and synthetically construct intercellular circuits with immune-like functions to understand how circuit architectures empower immune behaviors such as threat detection and cancer elimination while maintaining the ability to return to a non-inflammatory state. This work promises to uncover the “design principles” underlying various immune functions, providing a foundation for engineering novel immunotherapies. Dr. Dhiraj received his PhD and MS from Stanford University, Stanford and his BTech from Indian Institute of Technology, Roorkee.
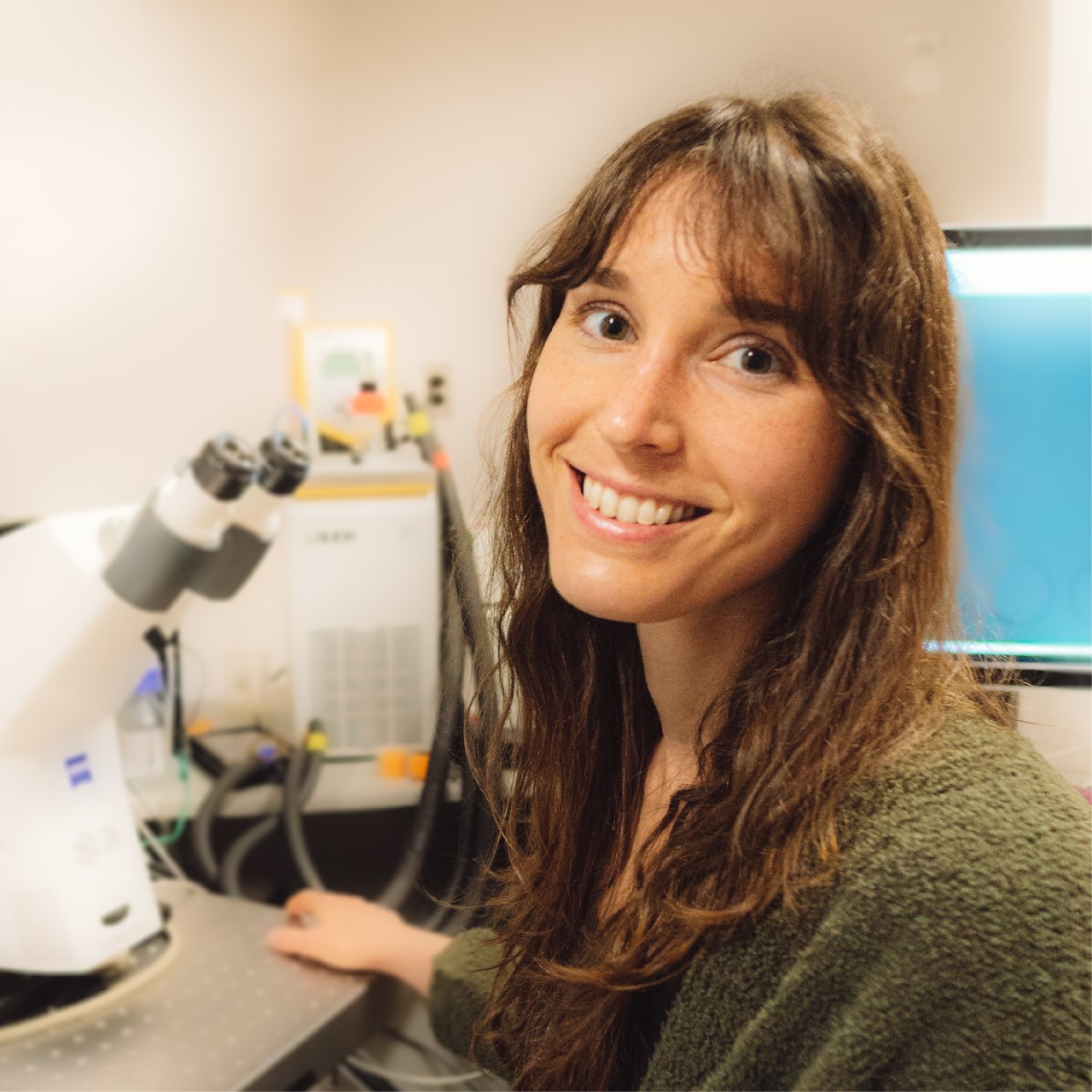
Every cell contains specialized compartments called organelles that perform distinct functions, and cells employ counting mechanisms to finely tune organelle population. Centrioles are one type of organelle required for proper cell division and mammalian development. Cells normally contain two or four centrioles, depending on cell cycle state, and centriole gains or losses result in cancer. One exception to this rule are the cells that line our airways, brain ventricles, and reproductive tracts. These cells contain hundreds of centrioles-yet how these specialized cells break the rules of conventional cell cycle-regulated counting mechanisms remains a mystery. Dr. Jewett's [Merck Fellow] work utilizes primary cell culture and in vivo models to understand the molecular framework that allows increased numbers of centrioles in certain cell types. This work will advance our understanding of how defects in centriole growth cause human diseases such as cancer. Dr. Jewett received her PhD from the University of Colorado School of Medicine and her BS from the University of Denver.
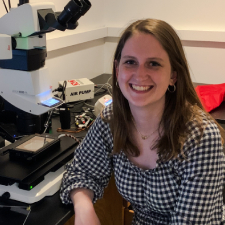
Dr. Johnson [HHMI Fellow] studies the role that a particular type of cell-cell communication, known as quorum sensing, plays in the development of spatially structured bacterial communities called biofilms. Biofilm formation promotes disease in many clinically relevant bacterial species, and infections caused by them pose severe risks for patients receiving chemotherapy. Dr. Johnson is currently investigating how quorum sensing within biofilms establishes patterns of gene expression, and in turn, how these patterns drive biofilm development and dictate biofilm architectural features. By defining mechanisms underlying biofilm formation and biofilm architecture, Dr. Johnson hopes to contribute to the generation of new approaches for disrupting quorum-sensing-controlled bacterial community interactions as a means of combating bacterial pathogens. Dr. Johnson received her PhD from MIT and her BS from Yale University.
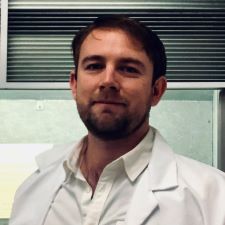
Cells are compartmentalized into membrane-bound and membrane-less organelles, providing spatial structure to the cell’s concentration of proteins and nucleic acids. Dr. Kilgore’s research aims to understand the environment inside different organelles and apply this knowledge to the development of targeted cancer therapies, as better targeting within the cell will improve drug efficacy, increase potency, and decrease side effects. Using both live cells and reductionist models, he will investigate how molecules distribute themselves within the cell as a function of their chemical properties. Learning and applying the chemical grammar of this spatial partitioning will enable the design and preparation of molecular probes and drugs that synergize with the chemistry of the cell as a mechanism of treating all cancers. Dr. Kilgore received his PhD from Massachusetts Institute of Technology and his BS from the University of California, Berkeley.
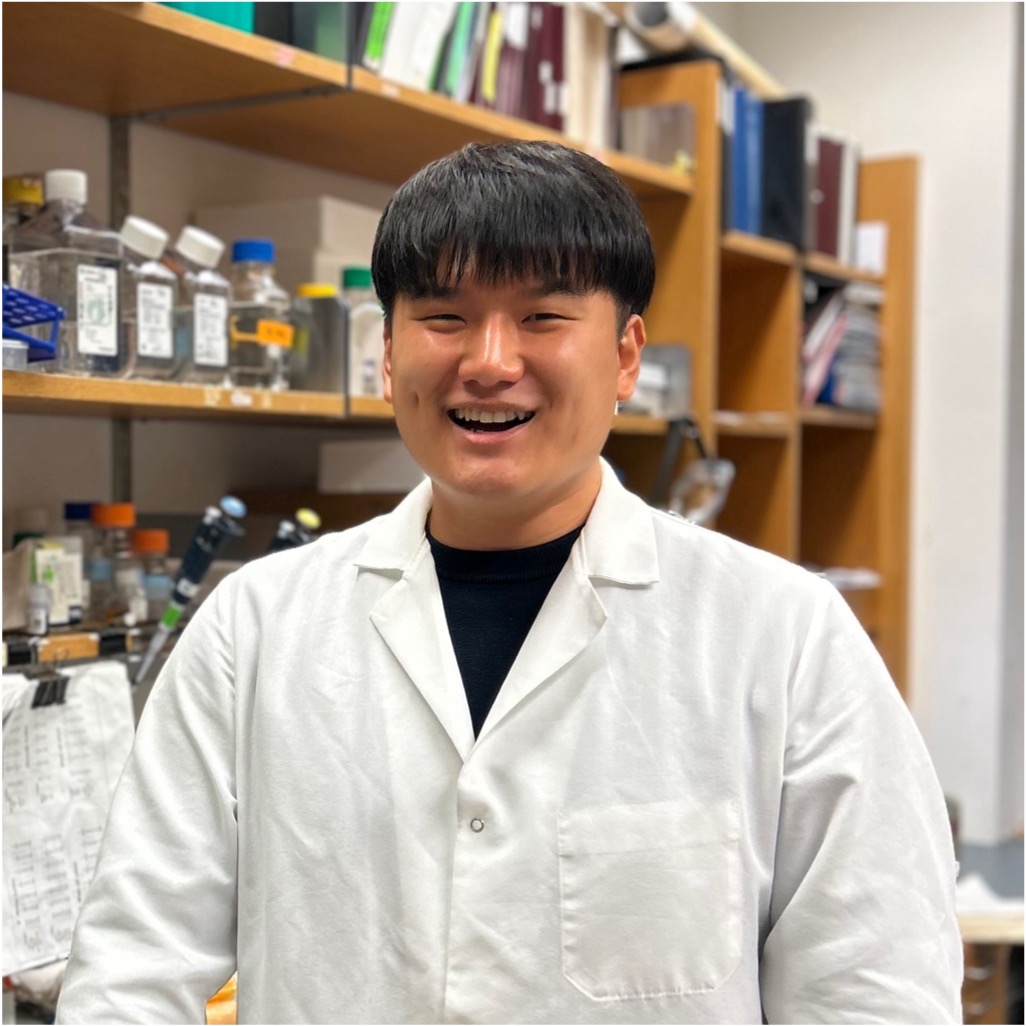
The cellular response to DNA damage is coordinated by an enzyme known as ATM kinase. Mutations in ATM are found in approximately 1% of the population and contribute to an increased risk of both hereditary and sporadic cancers, including breast cancer. Dr. Kim’s research investigates how ATM suppresses the production of double-stranded RNAs (dsRNAs) in response to DNA damage. These dsRNAs play a critical role in tumor progression. Dr. Kim aims to identify the key molecular players involved in ATM-mediated suppression of dsRNAs and elucidate how the loss of ATM function triggers inflammatory responses through dsRNA sensing pathways. By uncovering these mechanisms, Dr. Kim aims to deepen our understanding of how ATM mutations drive cancer development and uncover novel therapeutic strategies for ATM-associated cancers. Dr. Kim received his PhD and BS from the Ulsan National Institute of Science and Technology, Ulsan.

Radiopharmaceuticals, or drugs that contain radioactive forms of chemical elements, have transformed cancer diagnosis and treatment. Radioactive copper and manganese, for example, play a crucial role in PET imaging, while radioactive lutetium is used to deliver targeted radiation to cancer cells. While these radiometals have tremendous potential, however, their application is hindered by a lack of efficient “chelators,” or molecules that can securely bind radiometals in the human body. Computational protein design offers a solution by engineering protein-based chelators optimized for radiometal coordination, stability, and biocompatibility. Using advanced protein modeling, Dr. Klauser will develop chelators for radiometals, improving diagnostic imaging and advancing lutetium-based radiotherapies. While this work is applied to HER2-positive gastric cancer, these strategies have broad applications across various cancer types, ultimately enhancing precision oncology and expanding radiopharmaceutical utility.
This research develops a computational strategy to design stable, compact metal-binding proteins for radiopharmaceuticals, enabling fusion with therapeutic antibodies. Using diffusion models, such as RFdiffusion, thousands of protein backbones are generated for metals like copper and manganese. Sequences are assigned via ProteinMPNN, filtered for stability and binding with AlphaFold 3. For rare lanthanides, symmetric duplication of known binding motifs is used. This approach streamlines the discovery of stable scaffolds for radiopharmaceutical applications.